Extremophiles: defining the physical limits at which life can exist
By Jaqueline Ramirez
Understanding the origins of life and the first evolutionary events on Earth is an integral part of our quest to find life forms on other planets. For this, and although it is a complex concept, it is important to try to establish a definition of life (or, at least, a characterization of life) and to consider the limits and physical parameters in which it can develop.
Starting from a prevalent working definition of life accepted by NASA — that “life is a self-sustaining chemical system capable of Darwinian evolution” — in outer space the high solar incidence, extreme temperatures, altered gravity, and extreme concentrations of salinity and sulfates could decrease the availability of nutrients and make this a highly hostile environment for the proliferation of any organism.
Even so, the occurrence of microbial life that inhabit extreme inhospitable environments on Earth like deep sea hydrothermal vents, hypersaline systems, hot springs, halite crystals, sub-surface caves, rocks, and acid-mine drainages has been widely documented, defining the physical and chemical limits in which life as we know it here is possible and constituting a remarkable group of organisms that we call extremophiles.
Interest in extremophile organisms began in the mid-1960s when T. Brock and his collaborators isolated from Yellowstone National Park in the United States a filamentous bacterium capable of developing at high temperatures: Thermus aquaticus. Since then, numerous expeditions have been made to study the ecology, diversity, and physiological adaptations of these kinds of organisms (many of them microbial), as they are not simply tolerant of extreme physicochemical conditions, but actually require them to grow.
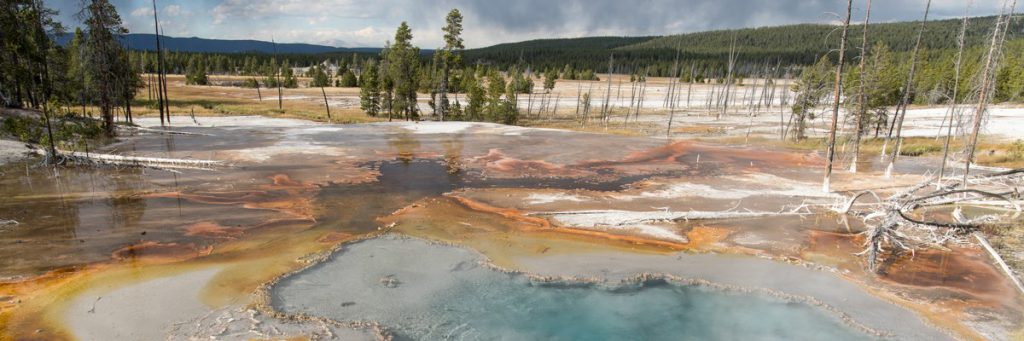
Extremophiles are classified according to their characteristics: hyperthermophiles, which tolerate temperatures of up to 122°C, have a name that implies “high temperature loving”; halophiles, which develop in brines, are “salt loving”; piezophiles, capable of growing at pressures of up to 1,100 atmospheres in the abyssal areas of the ocean, are “pressure lovers”; psychrophiles, which reproduce at temperatures below 5°C, are the cold tolerant organisms; there are the acidophiles, which live in highly acidic environments (with a pH lower than 5) or, conversely, alkaliphiles, capable of living in very alkaline environments (with a pH greater than 9); and there are also oligophiles — organisms that survive in low concentration of nutrients.
Since most of the ecological habitats of extremophiles on Earth have resemblances to some environments that have been present on some other planetary bodies in terms of biogeochemistry, nutrient composition, or topological similarities, the exploration of extremophiles on our planet is critical to identifying novel biosignatures that can be used in exploring habitable environments beyond Earth. In this context, depending on the planetary body, different (poly)extremophiles could persist. For example, halopsychrophiles might be able to persist in the subsurface oceans of worlds like Titan and Europa, and also on Mars which could have Cl-rich subsurface brines. Some such lifeforms may also need to withstand high pressures, given that, for example, the hydrostatic pressure of the potential subsurface ocean at Titan is estimated ranges from 140 to 800 MPa (see Fig. 1) and such pressures are beyond the range of the most extreme cultured piezophile on Earth (Thermococcus piezophilus; Pmax = 125 MPa).
For this reason, whether or not other planetary bodies such as Mars, Enceladus, or Europa could or did support life, future research will need to focus more on the interaction factor between multiple physicochemical parameters.
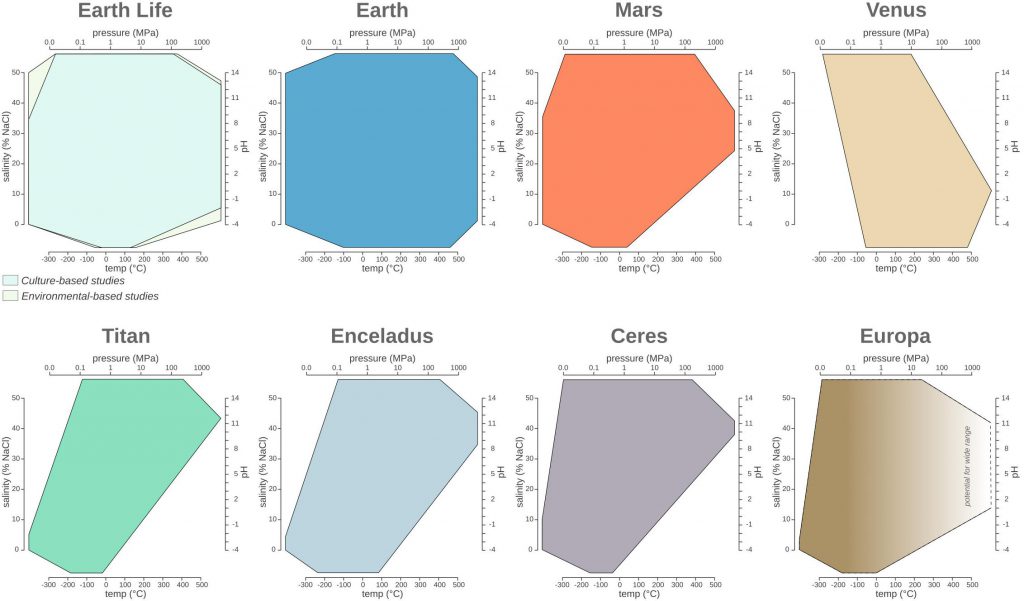
References:
- DasSarma, S., Schwieterman, E.W., 2021. Early evolution of purple retinal pigments on Earth and implications for exoplanet biosignatures. International Journal of Astrobiology 20, 241–250. https://doi.org/10.1017/S1473550418000423
- Lammer, H., Bredehöft, J.H., Coustenis, A., Khodachenko, M.L., Kaltenegger, L., Grasset, O., Prieur, D., Raulin, F., Ehrenfreund, P., Yamauchi, M., Wahlund, J.-E., Grießmeier, J.-M., Stangl, G., Cockell, C.S., Kulikov, Y.N., Grenfell, J.L., Rauer, H., 2009. What makes a planet habitable? Astronomy and Astrophysics Review 17, 181–249. https://doi.org/10.1007/s00159-009-0019-z
- Merino, N., Aronson, H.S., Bojanova, D.P., Feyhl-Buska, J., Wong, M.L., Zhang, S., Giovannelli, D., 2019. Living at the Extremes: Extremophiles and the Limits of Life in a Planetary Context. Frontiers Microbiology 10. https://doi.org/10.3389/fmicb.2019.00780
- Thombre, R.S., Vaishampayan, P.A., Gomez, F., 2020. Applications of extremophiles in astrobiology, in: Physiological and Biotechnological Aspects of Extremophiles. Elsevier, pp. 89–104. https://doi.org/10.1016/B978-0-12-818322-9.00007-1
Of Mexican origin, Jaqueline is a master’s student who is fascinated by the ancestral microbial lineages. Her main interests are Microbial Ecology and its relationship with Astrobiology.