The limits of human exploration: Problems and solutions to cosmic space travel
by Oliver Kimmance
Introduction
The concept of traveling between stars throughout the universe has been envisioned by humanity for thousands of years. Until recently, this has purely been through our imagination, but with recent leaps in technological development in the last 50 years, this notion has turned from fiction into a real and exciting possibility. Not only this, interstellar space travel is becoming increasingly necessary as our pursuit to find and understand extraterrestrial life grows exponentially, and as we uncover more information about the finite resources and environmental problems we face here on Earth.
In the unlikely absence of any major natural disasters, it has been theorised that Earth can continue to support life for another 1.75 billion years (Parry, 2013). This figure arises from the fact that around that time our Sun will have exhausted a considerable amount of its hydrogen fuel in a process called nuclear fusion, reducing its mass but releasing more and more energy in the form of solar radiation. This will dramatically alter the Goldilocks Zone of our solar system (the region in which liquid water can stand at the surface of a planet based solely on orbital characteristics), with Earth being withdrawn from this zone and becoming increasingly too hot to support life as we know it after approximately 1.75 billion years. You may think this is a huge range of time for humanity to leave Earth and settle in other solar systems, and you would be correct, however it also assumes that Earth is not made uninhabitable by other factors such as a large planetary impact or runaway climate change within that time frame.
Either way our time on planet Earth is finite, making it inevitable that in the near or distant future we must settle on other planets and even other star systems in the Milky Way. This of course requires transporting large numbers of people across vast interstellar distances (at least assuming we maintain our current physical form), a task unsurprisingly met by numerous problems.
Problems with interstellar travel: Current technologies
Interstellar distances are of course immense, but it can be difficult to fathom quite how immense. Travelling to our closest other star, Proxima Centauri, would be equivalent to travelling to and back from Pluto at its furthest distance from us in its orbit 2667 times. Even light, the fastest travelling entity in the universe, takes 4.24 years to reach us here on Earth from Proxima Centauri.
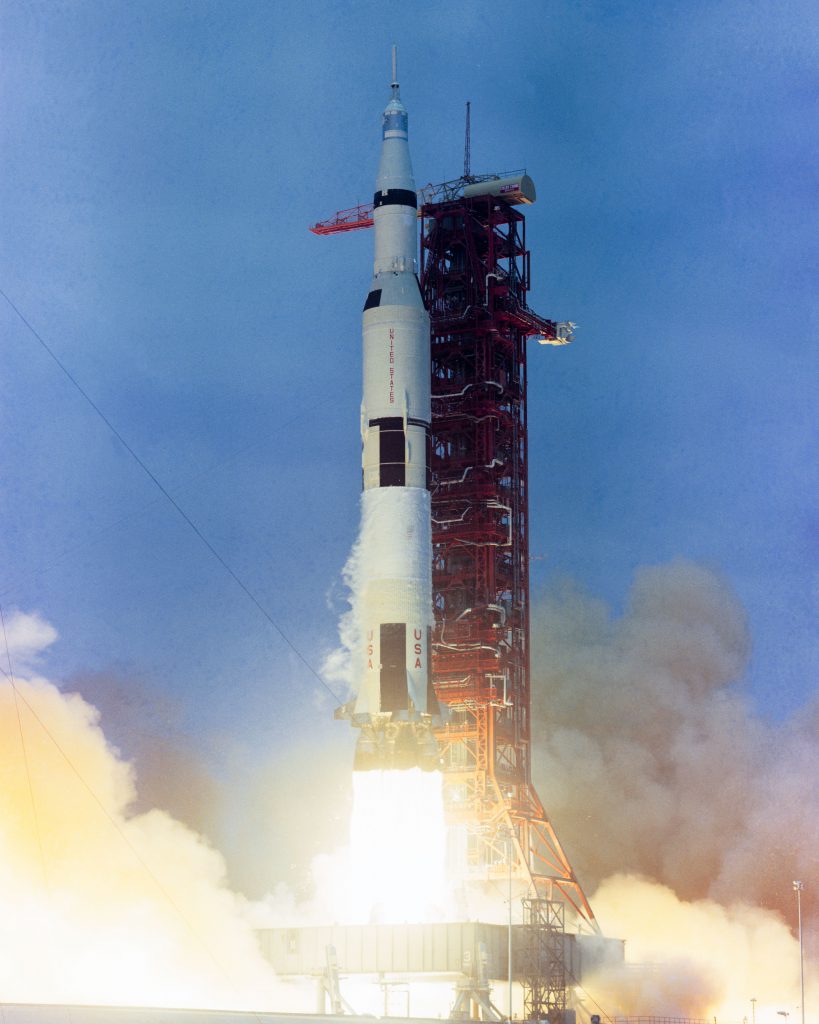
Unfortunately, current spacecraft technologies simply cannot travel such astronomical distances in feasible time frames, especially not when carrying the weight of hundreds of people to settle in other solar systems. Even using the fastest ever crewed spacecraft, Apollo 10, which travelled at approximately 40,000 km/h, it would take over 115,000 years to complete the journey to Proxima Centauri. To put this into perspective, the Homo sapiens species only developed speech as a cognitive function around 50,000-150,000 years ago, so the time it would take the generations of humans on the spacecraft to reach their destination would be equivalent to the time taken for the entirety of human verbal communicative history to play out.
In the confinements of a spacecraft with little to no selective pressures (except perhaps the ability to cope in such an environment), and with the ever increasing time taken to send and receive transmissions with humans back on Earth, the rate of adaptation and evolution of people on such a mission would dramatically decrease. Provided humanity back on Earth avoided any major disasters and continued to learn and develop new technologies, hypothetically, by the time such a crew reached Proxima Centauri they may be as underdeveloped compared to humans back on Earth as cavemen are to us right now! This is a disconcerting thought, but it highlights the necessity for new ideas and faster space travel if humanity is to leave Earth and venture into other solar systems.
All rockets used to date have been powered by chemical-based fuel which is heavy to carry and extremely inefficient. Chemical fuels exploit the energy released from the rearrangement of bonds during chemical reactions to generate thrust and accelerate the spacecraft. However these types of reactions only rearrange the electrons in atoms which for hydrogen, the predominantly used fuel source, makes up a mere 0.05% of the atom’s total mass. As explained by the equation for mass-energy equivalence from Einstein’s General Relativity: E = mc2, the smaller the mass involved with an interaction, the less energy is released. This can be used to calculate the efficiency of chemical fuel, coming in at a measly 0.0001% (Siegel, 2020).
Evidently we require the development of more efficient sources of power that can accelerate spacecraft to much faster speeds than is currently possible, in order to reduce the travel time of interstellar missions to a practical length. Fortunately, there are multiple exciting new technologies under development, and many more theoretical solutions that with increased scientific information may become feasible in the near future.
Potential new technologies
One exciting new concept is nuclear fusion fuel. Nuclear fusion has been recognised for some time, it is the process that occurs in the core of the Sun and other stars as the incredibly high temperatures achieved as a result of immense gravitational pressure causes small atoms such as hydrogen to fuse together and form helium and larger atoms, releasing energy in the process. Using nuclear fusion fuel to power spacecraft attempts to replicate this process, but the temperatures required to initiate these reactions are extremely hard to reach without the immense pressures achieved in the centres of stars due to their gravity. Consequently, new methods are required to stably heat hydrogen to the temperatures required for nuclear fusion reactions to take place.
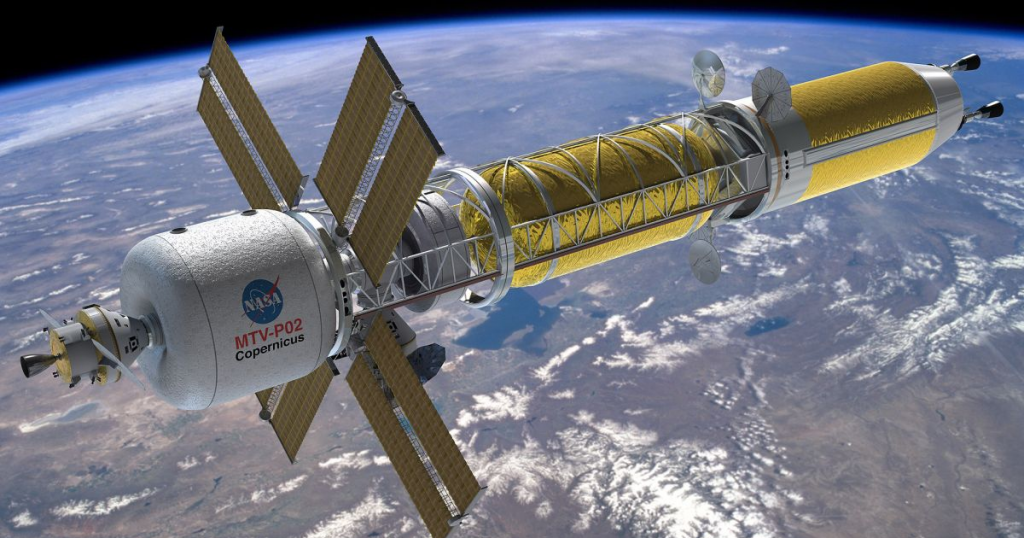
One such method is the ‘ITER magnetic confinement reactor’ currently under development in France. A circle of enormous electromagnetic superconductors generate enough pressure to squeeze a central ring of hydrogen gas into a plasma, allowing their nuclei to fuse into helium and generate energy. However, to prevent the superconductors vaporising themselves, a network of tubes containing liquid helium cooled to -270°C must surround the superconductors to stabilise the system. This requires huge amounts of energy to maintain and currently the energy input to carry out the reaction is more than is obtained from nuclear fusion.
Nevertheless, continued improvements to this technology could approve nuclear fusion as a more efficient and powerful means of powering the next generation of spacecraft. Compared to chemical-based fuel which utilises only 0.05% of the reagents total mass, nuclear fusion reactions rearrange the proton and neutron nuclei of atoms, entities with significantly more mass than elections and subsequently producing around 10,000 times more energy per unit mass of fuel. Spacecraft equipped with nuclear-based fuel could therefore be accelerated for much longer periods of time and achieve much faster speeds than current spacecraft. Furthermore, the only reagents necessary for hydrogen nuclear fusion are hydrogen isotopes, the most abundant elements in the universe. Theoretically, future spacecraft using this technology could gather cosmic hydrogen as they travel, feed the hydrogen into the reactor, and continue generating thrust. This would also reduce the amount of initial hydrogen required to power the spacecraft, greatly reducing its mass and therefore allowing it to be accelerated to greater speeds.
Another proposed method for dramatically increasing the speed of spacecraft is laser-powered propulsion, well known for being used in the ‘Breakthrough Starshot’ program. This revolutionary technology removes the need for on board fuel since the energy is provided from a ground based source. This dramatically reduces the weight of the spacecraft allowing it to be accelerated to much faster speeds than previously possible. A ground-based array of high power lasers all fire a beam into space that converges on a huge reflective light-sail attached to the spacecraft in orbit to accelerate it. To be effective, the mass of the entire spacecraft must be in the order of grams, whilst the combined energy output of the laser array must be in the order of hundreds of Megawatts.
Nevertheless, the Breakthrough Starshot program predicts that their ~1 gram probes can be accelerated to around 20% the speed of light, an incredible achievement that would reduce the travel time to Proxima Centauri down to only 22 years. This is more than a 5000-fold decrease in travel time compared to the fastest crewed spacecraft to date, and would allow the mission to be completed and data to be returned to Earth well within a human’s lifetime. The success of a mission like Breakthrough Starshot would be invaluable for gaining information on other solar systems and potentially habitable exoplanets, and would be an immense achievement, however a 1 gram spacecraft is clearly insufficient for transporting people across interstellar space in the eventuality of settling on worlds around other stars.
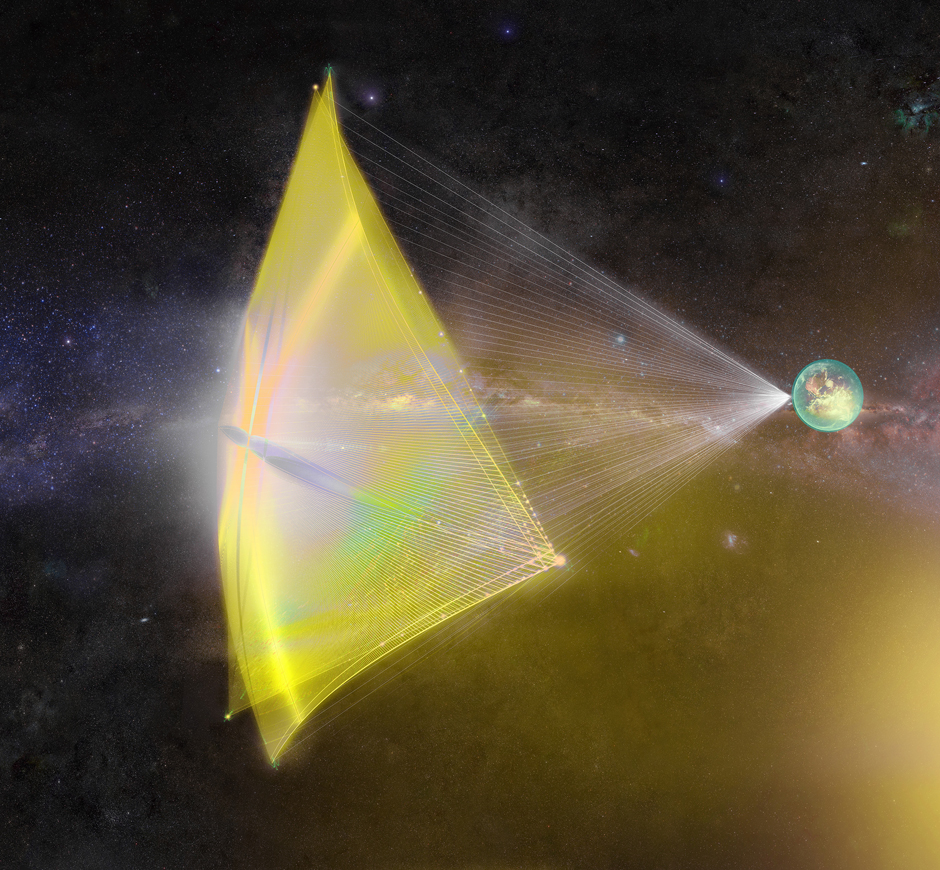
The obvious problem of scaling up the technology to power a spacecraft capable of accommodating passengers on its journey is a massive engineering dilemma, and perhaps is not possible. This is coupled with the fact that there is also no current solution as to how human passengers would survive the force experienced when traveling at such speeds. One problem will be the construction of a light-sail large enough to reflect enough energy to power a spacecraft many orders of magnitude heavier than those currently under development, but the most difficult problem will be providing the amount of energy required in the first place. Let’s say we wish to accelerate a spacecraft to 20% the speed of light with the mass of Apollo 10 (28,834 kg), bearing in mind this had only 3 crew members. The energy required to accelerate the Breakthrough Starshot probe to this speed is around 100 MW so the energy required to accelerate this manned spacecraft to the same speed would be 2.9 million GW, almost double the entire energy consumption of India in a one hour period . Evidently this amount of energy usage is far from possible, however there are theories for future energy harnessing methods that may bring this into the realms of possibility. Such proposals include construction of a Dyson sphere or harvesting cosmic hydrogen for fuel cells, but are beyond the scope of this article.
Further problems with laser-beam propulsion include collision of the spacecraft with interstellar dust particles, which would completely destroy the lightweight light-sail at such high speeds. Breakthrough Starshot will attempt to evade this by propelling thousands of probes in the hope that at least a few reach Proxima Centauri undamaged.
Limitations of intergalactic space travel
The prolonged future survival of humanity noticeably depends on the settlement of other planets and eventually other star systems in our galaxy. Accomplishing this would be a tremendous achievement and would allow humanity to expand and develop exponentially as the raw materials from more planets and the energy from more stars could be harnessed. However, unfortunately there seems to be a physical limit to the extent of human expansion that is built into the physics of space and time.
At the instant of the Big Bang 13.8 billion years ago the universe was a tiny pocket of energy, with high density and low density regions due to quantum fluctuations. Moments after the big bang, cosmic inflation stretched these sub-atomic density differences into enormous differences, millions of light years across in distance. After this event, gravity began to combine the more dense regions back together, forming clusters of galaxies, whilst the less dense regions had insufficient mass for gravity to prevail and so continued expanding. The result was the formation of millions of clusters of galaxies, all separated by expanding space. This also means that we are only gravitationally bound to our ‘local group’, a cluster of galaxies including our Milky Way, the Andromeda Galaxy, and around 50 dwarf galaxies, whilst all other clusters of galaxies are moving away from us as a consequence of the expansion of the Universe. Well, technically these superclusters are not moving away from us themselves, rather the space in between us and them is expanding and so the relative distance is increasing. Regrettably, this means that even if we could travel near the speed of light, the local group is likely to be the only area of the Universe humanity will be able to explore.
Moreover, as a result of dark energy, a phenomenon not officially discovered but whose effects can be observed, the expansion of the universe is accelerating. The further and further away we look into space, the faster and faster those galaxies and superclusters are moving away from us. This provides us with a ‘reachable zone’, much like a black hole event horizon where anything that passes beyond this horizon is traveling faster relative to us than the speed of light and is therefore unreachable and impossible to interact with. Earth’s ‘reachable zone’ is approximately 18 million light years in radius and makes up only 6% of the observable universe, comprising our local group and many other clusters that are accelerating away from us but at a slower rate relative to us than the speed of light. This can be a discouraging statistic, but the reachable zone still contains billions and billions of stars and probably even more planets to be explored.
Theoretical solutions to intergalactic space travel
There are even hypothetical means permitted by our current laws of physics that may make it possible to travel faster than light, making the unreachable universe theoretically reachable. Two dominating and exciting theories are wormholes and warp drives.
Wormholes are hypothetical phenomena that form when two extremely dense entities such as two black holes distort and bend the fabric of spacetime so much that a tunnel between the two is formed. This may be between two points in space or even between two points in parallel Universes. There is currently no evidence of wormholes existing, but Einstein’s theory of general relativity allows for their existence. Unfortunately even if wormholes were discovered, it is also theorised that any matter passing through the wormhole, even a single particle, would trigger catastrophe and cause the wormhole to break down. One theoretical solution to this is to feed antimatter into the wormhole along with whatever matter is attempting to pass through, which would potentially stabilise the effects of regular matter passing through.
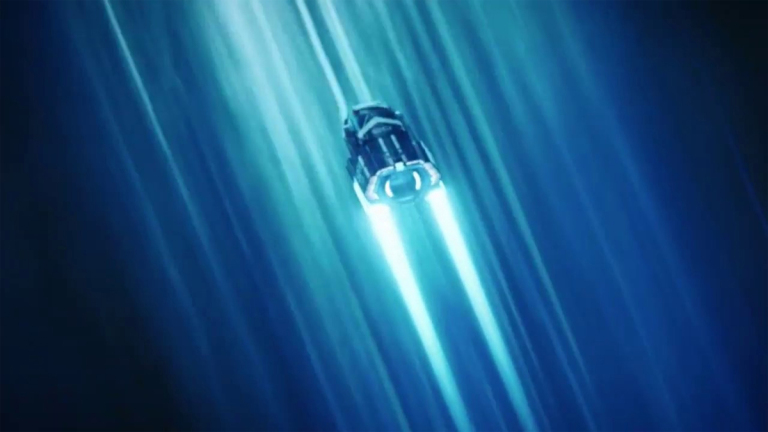
The concept of warp drives similarly plays on our understanding of space-time given by general relativity. This is a theoretical technology that would be attached to future spacecraft and distort space-time in front of the craft, massively reducing the distance between the spacecraft and its destination. By cleverly and carefully exploiting large quantities of matter and antimatter, space-time could be shortened in front of the spacecraft and lengthened behind it, creating a stable bubble of space surrounding the spacecraft and allowing it to travel to a particular destination far quicker than the speed of light.
Evidently, these two potential means of traveling faster than light are far from plausible at the present date, but the fact that they are both possible under our current laws of physics is extremely exciting. Perhaps with future discoveries and information gained on dark energy, dark matter, antimatter, and black holes, these models will become real-world technologies and allow humanity to freely explore the universe!
Oliver Kimmance is a Research Associate with the Blue Marble Space Institute of Science and is an undergraduate student in Biochemistry at the University of Bristol, UK.