How a Physicist Tackles Astrobiology
By Michael May
Introduction
If scientific research was reflective of high school level science courses, then every field would lie within its own separate sphere: biology dealing with all things living, chemistry describing the interactions of physical matter, and physics covering topics such as motion and the behavior of particles. However, it is fairly common knowledge that there is plenty of overlap between these fields, with studies such as biochemistry and biophysics dedicated to describing how one subject operates within the sphere of another. Approaching research and scientific questioning in this more integrated manner often leads to new insights that help scientists develop an even better understanding of natural phenomena.
But when is it useful to use one field to describe another? How do I as a physicist apply my knowledge to bring understanding to another field such as astrobiology?
The Scientific Question
My research group in the Young Scientist Program at BMSIS is looking into different possible mechanisms that could support life somewhere else in the Universe, starting first with how an organism might survive with a modified version of photosynthesis (used on Earth by organisms to harvest sunlight for energy).
The biological approach can give a broad perspective to the process and allow us to ask some interesting questions, such as:
- How might the biological structures differ to generate energy such as in the molecule ATP (adenosine triphosphate, also known as the energy currency of life as we know it)?
- How might different biological molecules affect the end result?
Meanwhile, an approach from chemistry can be even more specific:
- How effective can different solvents or elements be compared to water and carbon used by life on Earth? (All of life as we know it relies on water as a solvent for chemical reactions and uses carbon-based chemistry)
When looking at the physics of photosynthesis, there are plenty of ways to analyze how laws from fields such as quantum mechanics and electricity play an important role in the process, and can greatly expand upon the overall picture. However, when faced with a broad topic such as alternative biological mechanisms, trying to investigate ways that physics could operate at the particle and molecular levels within the cell would require a sufficient amount of knowledge in biology and chemistry. This would be to ensure that any changes in the physical operation can be supported by structures and chemicals that do not diminish the effectiveness of photosynthesis.
If looking at the physics of photosynthesis within the cell is too complicated for the project at hand, then how can a physicist apply their knowledge to a topic that is heavily entrenched in the ideas of biology and chemistry? The answer I found came from a mindset I adopted when first beginning my research: look for the parts that mostly or only rely on the principles of physics and apply that knowledge to the problem.
The Transfer of Energy
The first step of photosynthesis as described in biology is the absorption of light from the Sun, where that energy is transferred to electrons within chlorophyll that are used to power the subsequent processes. A broad generalization of biology that many people learn about does not describe the process of light absorption in more detail than that, but the properties of light and energy in this beginning step can be greatly described by the laws of physics.
For example, questions can be asked about the amount of energy that the photons being absorbed have, which can be calculated given the type of light (such as red, blue, etc.) that is absorbed. This energy can be transferred to electrons within the atoms of chlorophyll by exciting them to higher orbital levels, much like an elevator taking a person from the ground to a higher floor, which is described by quantum theory. The energy needed for excitation must be exactly equal to the difference in energy between two levels, which is why green light is reflected as it does not have the exact energy to excite an electron to a higher level; this reflection is what causes plants to appear green. Red and blue light offer just the right amount of energy to excite the electrons to a higher level, one could think of the story of Goldilocks as a good analogy to the excitation of electrons (not too hot or cold, but just right).
With this framework in mind, I can research alternatives to how energy is transferred to cells without being constrained by the known laws of biology and chemistry. By assuming that the mechanisms of photosynthesis are unchanged within the cell beyond the excitation of electrons, my focus can be narrowed to a pure physics problem about how to get energy from outside the cell to inside.
The Big Idea + Conclusions
At the time of writing this article, the current idea of energy transfer that I am working on focuses on the creation of secondary electrons from ice.
Just as photons can energize electrons in chlorophyll, there exist high energy “primary” electrons and other particles, which can be found in the magnetic fields of gas giant planets, cosmic rays (from stars), or from the decay of radioactive materials, that can strike an ice layer on a planet/moon and liberate a cascade of “secondary” electrons that were held to atoms in the ice through energy transfer. These secondary electrons of a lower, biologically conducive energy can travel down through the ice to another layer holding potential microorganisms. Perhaps within such a region, the cells could utilize the energy for further photosynthetic processes as seen below.
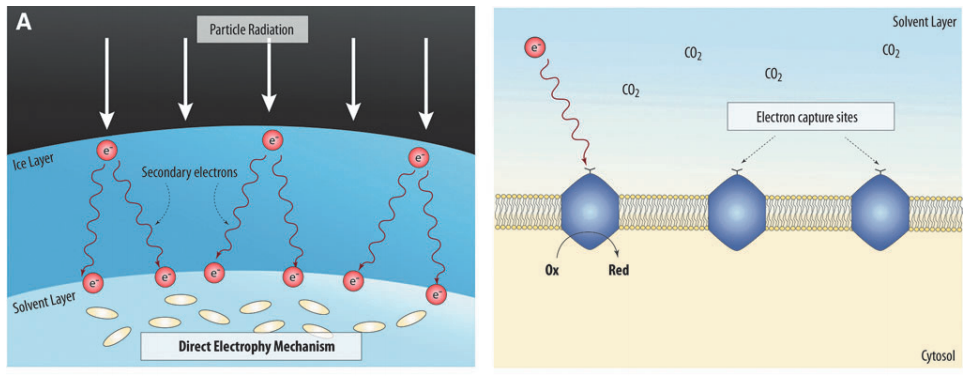
From there, some members in my team are researching how these microorganisms might behave under this ice layer and how their biology might function given the circumstances of their location. Others research possible sources of the incoming particle radiation and whether these sources could be viable, given how many secondary electrons they would need to produce to sustain biological life. Through this collaboration, a final picture of our research can be realized and result as a finished paper that is presented to the scientific community.
So what are the key takeaways from this? When I began this research project, it was one of the first instances where I worked with others who specialized in different fields other than my own and on a topic centered around a field I have not studied in years. From what I have learned about researching astrobiology, it is all about finding ways to utilize your knowledge in the given framework. Deconstructing the process of photosynthesis and analyzing where physics plays an important role has led me to find my path moving forward in the project.
From finding applications for your knowledge to collaborating with your partners, being able to find your role in the group and combine your knowledge with that of others are the key components to a successful research project.
References
- https://www.liebertpub.com/doi/abs/10.1089/ast.2016.1510?journalCode=ast
- https://www.sciencedirect.com/science/article/pii/S0005272811001782
- https://www.sciencedirect.com/science/article/pii/027311779390068M
Michael May is an undergraduate at the University of California, Berkeley majoring in astrophysics and physics and is a Research Associate for the BMSIS Young Scientist Program. He is working with Dr. Dimitra Atri on different theoretical mechanisms for life to exist in the Universe.